2 The 4 Technology Solutions
EFFECTS FROM TEMPERATURE CHANGES
An On-Line Version of a Column First Published in: Environmental Technology May/June 1996 Vol. 6 No. 3 Pages 24-25
by: David B. Vance dbv7@mindspring.com
The previous Groundwater Column examined the overall transport of heat in saturated groundwater systems. This column will examine the effects of temperature on physical, chemical, and biological processes that govern the transport and degradation of contaminants in an aquifer.
First physical effects, the two dominant physical characteristics of groundwater that change with temperature are viscosity and density. The figure illustrates those changes over the temperature range of 0oC to 100oC. Most striking is that those curves are not linear. In the temperature range of 0oC to 30oC, the viscosity decreases by over 50%. This change improves the efficiency of both advective fluid flow and diffusional transport. In contrast, water density decreases significantly at temperatures over 60oC.
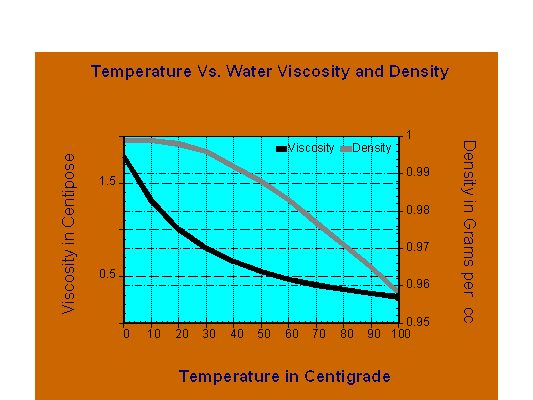
Effect of Temperature on Groundwater Viscosity and Density
Next chemical reactions should be considered. Most salts increase in solubility as the temperature increases, the common exception to this general rule being carbonates in which solubility decreases as temperature increases. As temperature increases the solubility of gases in groundwater decreases. The solubility (in mg/L) of environmentally important gases over the temperature range of 5oC to 35oC is as follows:
- Oxygen with air as the source 12.7 to 7.1;
- Carbon Dioxide 2,774 to 1,105; and
- Methane 34.1 to 17.3.
The relationship between temperature and the kinetics of chemical reactions is expressed by the Arrhenius equation:
ln k = ln A - Ea/RT
- Where k is the rate constant,
- A is an integration constant,
- Ea is the energy of activation,
- R is the universal gas constant, and
- T is the temperature in Kelvin.
Commonly this relationship is graphed as ln k vs. 1/T, which results in a straight line in which k increases exponentially as T increases. Under proper circumstances small increases in temperature can have large effects on kinetic rates.
In addition to reaction rates, temperature also effects equilibrium constants, particularly those that involve reactions with non-covalent bonds. Covalent bonds are relatively resistant to thermal perturbation. However:
are disrupted by relatively small temperature changes.
- Hydrogen bonds, ionic bonds and van der Waals interactions all form with the release of heat,
- hydrophobic interactions form with heat consumption.
- Increases in temperature will destabilize the first three,
- while it will stabilize the hydrophobic interactions.
As temperature increases the solubility of hydrocarbons increases, not uncommonly 2 to 3 times in the temperature range of 5oC to 30C. Through this and the bonding reactions mentioned above, the forces responsible for the retardation of hydrocarbons within the soil matrix are reduced as temperature increases, improving the flushing action of groundwater flow induced by pump and treat recovery systems.
Vapor pressure also increases with temperature, increased vapor pressure results in increased mass transport rates in soil vapor extraction systems. Which is where many of the prior applications of external heat to subsurface remediation have been used.
Following are examples of the percent increase in vapor pressure as the result of a temperature increase from 5oC to 35oC:
- Benzene 417%;
- Ethylbenzene 638%; Toluene 513%;
- TCE 476%; and
- Tetrachloroethylene 545%.
With regards to free product recovery, an increase in temperature will reduce the hydrocarbon viscosity and increase the recovery of free product from the surface of a water table. A temperature increase from 10oC to 50oC can increase the recoverable amount of middle distillates by 10%.
Molecular diffusion is driven by the presence of a chemical concentration gradient between adjacent zones in the subsurface. The presence of a temperature gradient will also set up the diffusion of dissolved components in a fluid. The phenomena is termed the Soret Effect and is known generally as thermal diffusion. Mass transport rates under thermal diffusion are described by the equation:
NAT = DT(Rho)d ln (T/dz )
- Where NAT is the mass transport rate as number of moles/cm2/second;
- DT is the coefficient of thermal diffusion in cm2/second;
- T is temperature in kelvins,
- z is the distance in the direction of diffusion in centimeters, and
- Rho is the fluid density in g-moles/cm3.
The magnitude of thermal diffusion is dependent upon the size and chemistry of the molecules involved as well as the temperature. At maximum it may reach a diffusional mass transport rate of 30% that seen for molecular diffusion driven by chemical concentration gradients, and it is typically significantly less than that.
Lastly, a brief discussion on temperature and biological systems. Bacteria typically have a relatively narrow temperature range (about 10oC) in which they experience maximum growth and metabolic activity. As groundwater temperatures increase the dominant bacterial consortia will change in response. This is true with increases of temperature up to about 35oC , temperatures of 50oC or above will cause traumatization and partially kill many bacterial species indigenous to the subsurface. It is common for the rates of physiological and biochemical processes to undergo a 2 fold increase due to a temperature change of only about 10oC. This is due to the nature of the Arrhenius equation previously discussed as applied to biological systems.
A practical note with regards to bench scale studies of soil bacteria, incubation of bacteria in the laboratory ideally should be conducted in a temperature range that is from 5oC below the in-situ groundwater temperature to 10oC above. On average the groundwater temperature in the United States is approximately 10oC. Most bench scale laboratory testing done is at room temperature (about 25oC).
In conclusion, many of the processes exploited in aid of subsurface remediation are impacted by temperature. The overall compounded physical/chemical/biological effects that can be anticipated within a relatively normal temperature range (5oC to 35oC) could on an additive basis make a 3 to 5 fold increase in remediation rates, which is not insignificant. The issue is then site specific, i.e. shallow vs. deep contamination. Passive heating systems using modification of the ground surface to improve heat adsorption or low intensity intrusive systems such as those associated with heat pump systems could offer remediation actions with possible economic benefits.
Return to the Environmental Technology Index Page
Copyright 2008 David B. Vance All Rights Reserved

If you have comments or suggestions, e-mail me at dbv7@mindspring.com
|